Here on Earth, life took hold very early on in our planet’s history — within the first few hundred million years at the latest — and has persisted ever since, surviving and thriving in an unbroken biological chain for over four billion years. Despite numerous rocky and icy worlds known in our own Solar System, as well as over 5000 known exoplanets orbiting stars other than the Sun, Earth remains the only instance where we’ve confirmed that life exists.
That doesn’t mean, however, that if we want to find life beyond Earth, we should restrict ourselves to searching for planets that are very similar to our own. Sure, they’re out there: Earth-sized worlds orbiting around Sun-like stars at similar distances to the Earth-Sun distance. But it’s an overly restrictive assumption to conclude that planets like ours are the only places where life arises.
In fact, Earth-like planets might not even be the best places to search for extraterrestrial life. In the great cosmic lottery of life, we don’t know:
- what the other prizes are,
- what the odds of winning any sort of prize are,
- and whether life on Earth is “the grand prizewinner” or whether there are still grander prizes out there.
In 2014, a pair of astrobiologists proposed the idea of a superhabitable planet: one with more suitable conditions for life’s emergence, evolution, and for greater biodiversity. Although many exoplanets have been touted as being super-habitable, the evidence is still murky. Here’s the science behind the idea of super-habitability.

If the light from a parent star can be obscured, such as with a coronagraph or a starshade, the terrestrial planets within its habitable zone could potentially be directly imaged, allowing searches for numerous potential biosignatures. Our ability to directly image exoplanets is presently limited to giant exoplanets at great distances from bright stars, but this will improve with better telescope technology.
Let’s be up front about the limitations of what we know. We know that the building blocks of life — from raw atoms to organic molecules to amino acids to water-rich rocky planets — are literally found all over the Universe. We even know how and where they occur.
Travel the Universe with astrophysicist Ethan Siegel. Subscribers will get the newsletter every Saturday. All aboard!
- A variety of processes, from nuclear fusion in stars to stellar cataclysms like core-collapse supernovae, exploding white dwarfs, and merging neutron stars, combine to create the full suite of elements making up the periodic table.
- In intergalactic gas clouds, in star-forming regions, in outflows from young stars, and in planet-forming disks around those stars, a wide variety of organic molecules continue to be discovered.
- In the inner regions of young stellar systems, as well as in asteroids and comets found in our own Solar Systems, a huge variety of complex molecules, including aromatic hydrocarbons and scores of types of amino acids, exist in great numbers and great variety.
- And all throughout the Universe, wherever stars exist, tremendous numbers of planets exist as well.
But not every star has planets, and not every planet is suitable for the development of life.

Although research from the early-2000s professed that habitability should only be possible in an annular ring surrounding most Milky Way-like galaxies, with low metallicity and frequent stellar cataclysms and/or dense gravitational interactions disfavoring life in the outermore or innermore regions, that research has been called into question, particularly concerning the inner galactic regions.
There were a number of missteps — i.e., assertions that were made early on that are now thought to be erroneous — that required astronomers to rethink what assumptions we ought to make when considering the possibility of habitability for an exoplanet.
We assumed, initially, that there would be a habitable zone: a region where a rocky planet with a sufficient atmosphere could maintain liquid water on its surface. We now know that many worlds outside of this so-called habitable zone could possess sub-surface oceans beneath a layer of ice, that exomoons could be habitable through tidal heating by a nearby planet, and that the right atmosphere could render an otherwise cold, barren world hospitable to life.
We assumed that having a Jupiter-like planet in our Solar System protected us from many major impacts; we now know that Jupiter actually increases the collision rate on Earth from asteroids and comets by something like 350%.
We assumed that all stars possessed a mix of terrestrial and giant planets; we now know that unless a star is rich enough in heavy elements, the formation of rocky planets cannot occur.
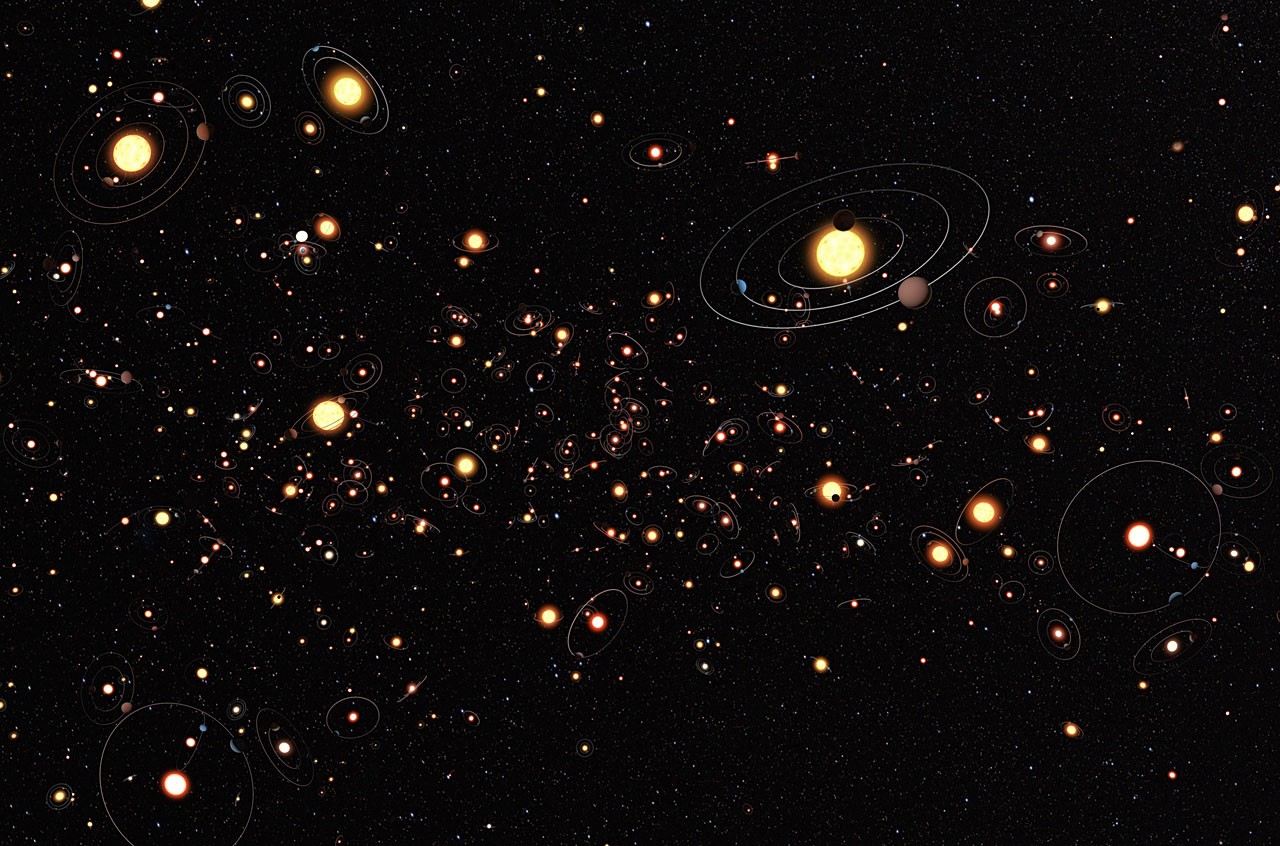
In dense environments with many stars, such as young star clusters, the galactic center, or the centers of globular clusters, gravitational interactions could perturb the orbits of exoplanets, rendering them unstable. However, this may not be the explanation as to why no planets have been found in globular clusters; perhaps the metal-poor nature of the clusters examined is why no planets are present.
And, perhaps most damningly, we assumed that super-Earths, or planets between 2 and 10 Earth-masses, were the most common type of planet in the Universe, and were for some mysterious reason nowhere to be found within our Solar System. Although it’s true that so far, among all the discovered exoplanets, there are more planets in this mass range than any other mass range, to categorize them as “super-Earths” is wildly misleading.
It turns out that when you measure the masses and radii of exoplanets together, you find that there are only three broad categories of exoplanet that exist.
- Terrestrial/rocky planets, which are typically no more than 120-130% the radius of Earth and no more than ~2 times the mass of Earth.
- Neptune-like planets, which have a thick, volatile gas envelope enshrouding their surface that’s at least thousands of Earth-atmospheres thick, which represent practically all of the so-called super-Earths up to planets with about the mass of Saturn.
- And Jovian planets, or gas giant worlds that exhibit self-compression, ranging from about 40% of Jupiter’s mass up to about ~13 times the mass of Jupiter, at which point the planet becomes a brown dwarf star, and above ~80 Jupiter masses, a full-fledged hydrogen-burning star.
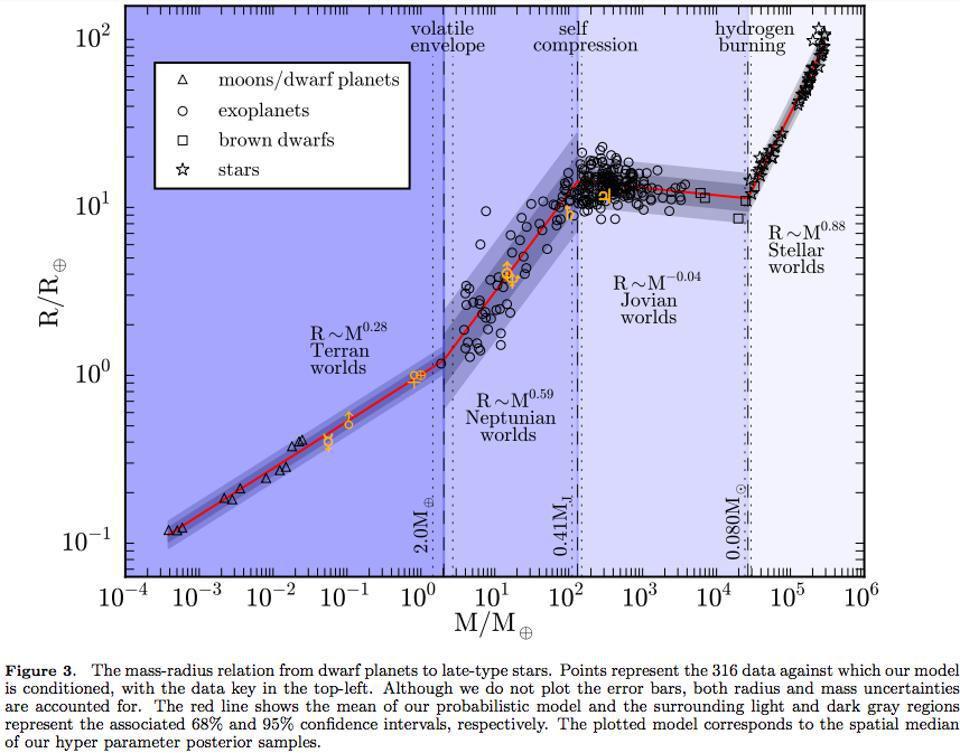
When we classify the known exoplanets by both mass and radius together, the data indicates that there are only three classes of planets: terrestrial/rocky, with a volatile gas envelope but no self-compression, and with a volatile envelope and with self-compression. Anything above that is a star. Planetary size peaks at a mass between that of Saturn and Jupiter, with heavier and heavier worlds getting smaller until true nuclear fusion ignites and a star is born. Saturn is just about the lowest density planet out there.
Yes, there are exceptions to these general rules, but the lesson is not to pin our hopes on these exceptions. Rather, the lesson is to search for the actual presence of life, as only once we actually have confirmation of life’s presence on another world can we begin to make intelligent statements about how likely a world is to harbor it.
In the meantime, declaring a world to be super-habitable is excruciatingly premature, as our notions of habitability are defined largely by our prejudices, not by data.
Nevertheless, there are a series of considerations that we should be making when assessing the conditions present on a planet in terms of habitability. We cannot be certain what set of conditions is more or less likely to lead to an inhabited planet, but we can be certain that these properties will affect the suitability of a planet for hosting life upon it. The details — which of course remain to be worked out — will require much more robust data than we presently have. As we think about the suitability of planets and planetary systems for life in the Universe, here are the top considerations we need to keep in mind.

This color-coded map shows the heavy element abundances of more than 6 million stars within the Milky Way. Stars in red, orange, and yellow are all rich enough in heavy elements that they should have planets; green and cyan-coded stars should only rarely have planets, and stars coded blue or violet should have absolutely no planets at all around them. Note that the central plane of the galactic disk, extending all the way into the galactic core, has the potential for habitable, rocky planets.
Metallicity. This is astronomer-speak for the fraction of heavy elements — elements other than hydrogen and helium — present in a stellar system. One of the most fascinating discoveries to come out of an analysis of the first 5000 (okay, 5069) exoplanets discovered is the fact that very few planets exist around stars that don’t have a solar-like abundance of heavy elements. Specifically, of all the known exoplanets with orbital periods of less than 2000 days (about 6 Earth-years):
- Only 10 exoplanets orbit stars with 10% or fewer of the heavy elements found in the Sun.
- Only 32 exoplanets orbit stars with between 10% and 16% of the Sun’s heavy elements.
- And only 50 exoplanets orbit stars with between 16% and 25% of the Sun’s heavy elements.
That means, all told, that only 92 out of the 5069 known exoplanets (just 1.8%) exist around stars with a quarter or fewer of the heavy elements found in the Sun. If you want to make a planet via the core-accretion scenario, which is the only way to make a rocky planet close to your parent star, you absolutely require enough heavy elements. There may be a “peak” in metallicity where life is most likely; beyond a certain abundance, life may become less likely again. The only way to know the metallicity-life dependence is to discover and catalogue systems with life on them.
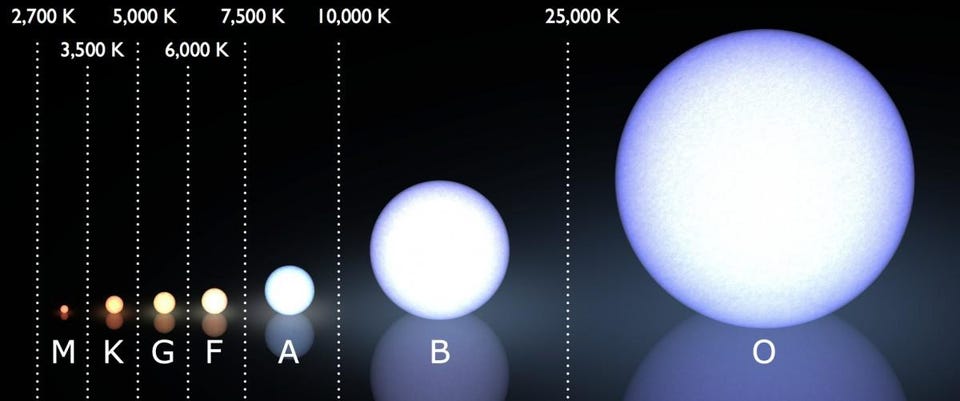
The (modern) Morgan–Keenan spectral classification system, with the temperature range of each star class shown above it, in kelvin. The overwhelming majority (80%) of stars today are M-class stars, with only 1-in-800 being massive enough for a supernova. Only about half of all stars exist in isolation; the other half are bound up in multi-star systems. Earlier on, when there were no heavy elements, virtually all of the stars that formed were O-and-B stars: the hottest, bluest, most massive type.
Star type. Here on Earth, we’re orbiting a G-type star: a star with one solar mass of material. Stars such as our Sun burn relatively stably for billions of years, increasing their energy output by a few percent every billion years. Once they pass the initial first few hundred million years, during which they flare copiously, they burn stably until evolving into a subgiant, a red giant, and then ending in a planetary nebula/white dwarf combination.
But our Sun is more massive than about 95% of all stars that exist. About 75-80% of all stars are low in mass: M-type red dwarfs. These stars are cooler, less luminous, and much longer-lived than our Sun. They flare more frequently, and all of their rocky planets quickly become tidally locked to them, where one side always faces their star and the opposing side always faces away. However, they also live for up to trillions of years, and burn at very stable luminosities, excepting their propensity for flares.
K-type stars are in between these two, and make up ~15% of stars: living longer than our Sun but without the flares of lower-mass stars. O, B, A, and F-type stars are all more massive and shorter lived than our Sun, but with greater energy outputs and lifetimes of up to 2-3 billion years themselves. Which star type is most conducive to life arising? It’s a smart question to ask; it’s a dumb question to pretend we have answers to.
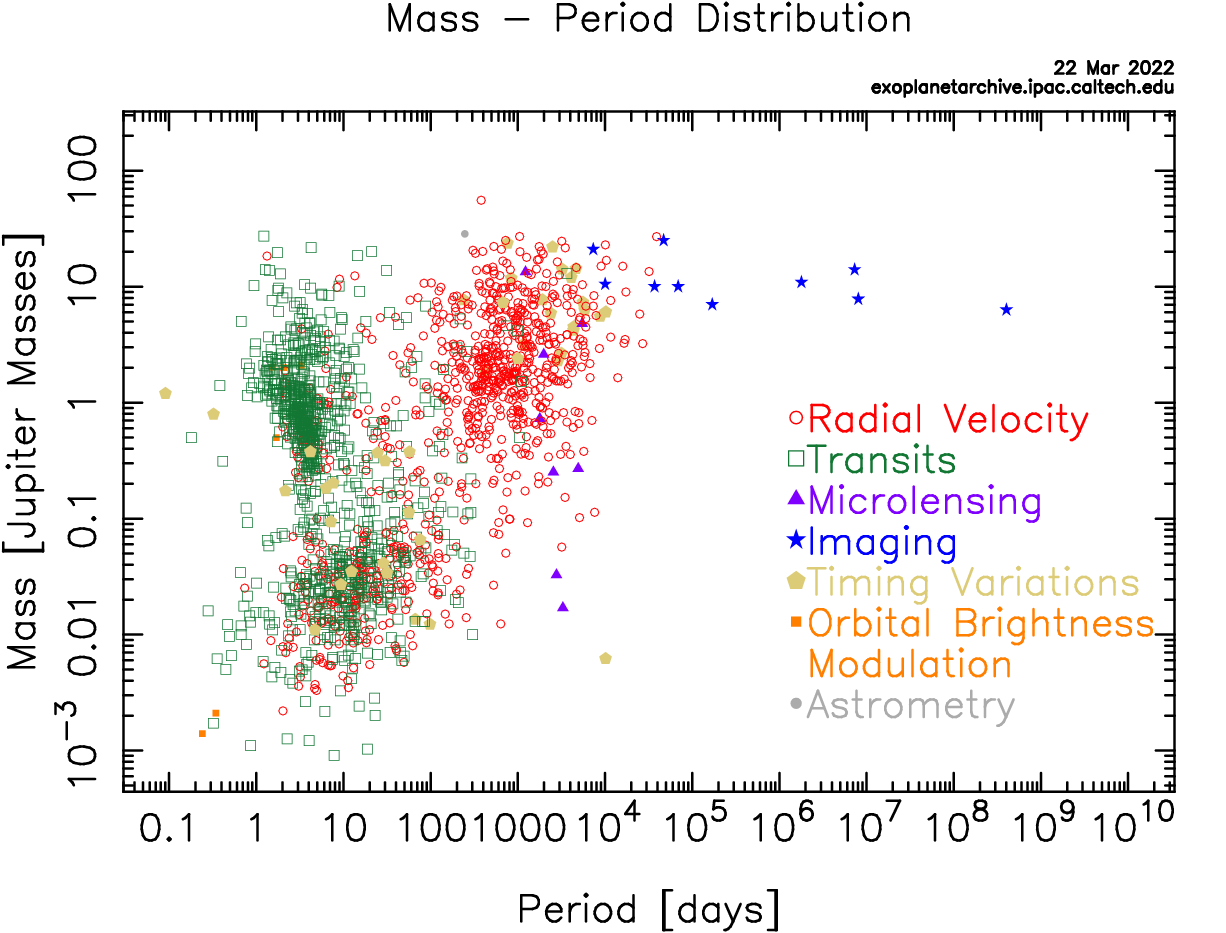
The mass, period, and discovery/measurement method used to determine the properties of the first 5000+ (technically, 5005) exoplanets ever discovered. Although there are planets of all sizes and periods, we are presently biased toward larger, heavier planets that orbit smaller stars at shorter orbital distances. The outer planets in most stellar systems remain largely undiscovered, but those that have been discovered, largely through direct imaging, are difficult to explain with the core accretion scenario.
Preferred planetary mass. Here’s a question for you: how great of a surface gravity is most preferable for life: Earth-like, less than Earth-like, or greater than Earth-like? How much surface area is the ideal, or most preferred amount, for life: more than Earth’s, less than Earth’s, or equal to Earth’s? What is the best land-to-water ratio for a planet to have in order to support life: mostly land, mostly (or exclusively) water, or some mix of land-and-water?
What about properties like a planet’s rotation rate: is slower or faster better?
What about properties such as axial tilt? Is large, small, or intermediate best? Does it matter if the axial tilt changes significantly over time — i.e., is it good to have a large, stabilizing moon — or is it inconsequential?
It’s easy to make grand statements at this point, because we have a total lack of evidence as to what conditions are most conducive to life. These are questions worth thinking about, especially as we start to understand the abundances of planets of specific masses around stars of specific classes, and their distributions in terms of these and other metrics. But until we have data on what fraction of planets with any specific set of properties are actually inhabited, all of this remains speculation.
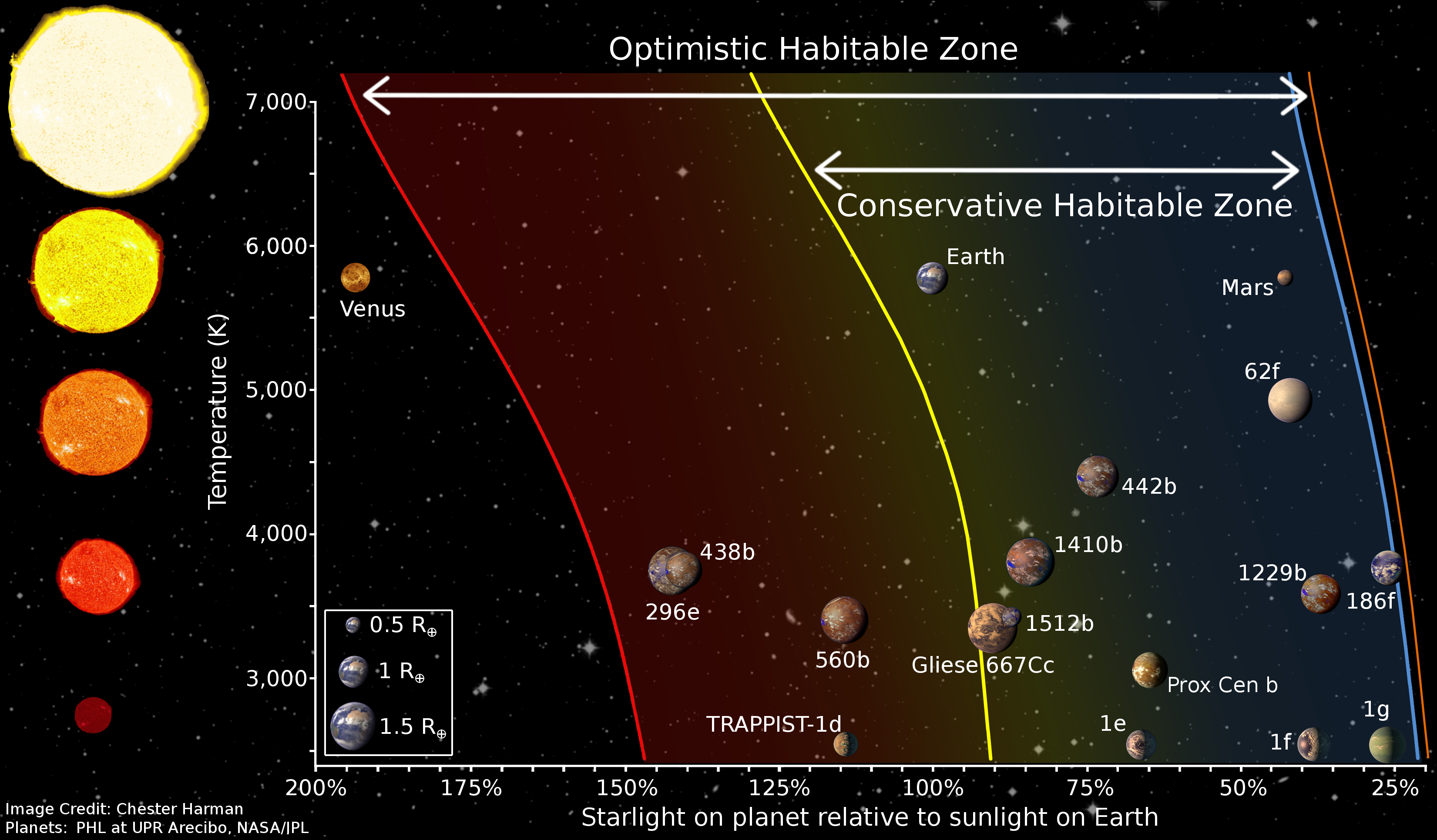
Our notion of a habitable zone is defined by the propensity of an Earth-sized planet with an Earth-like atmosphere at that particular distance from its parent star to have the capacity for liquid water, without a cover of ice, on its surface. Although this describes the conditions that Earth possesses, it is unknown whether this is a requirement, or even a preference, of life.
Since 2014, the prevailing hypothesis has been that the most massive but still rocky terrestrial planets would be the most likely to be inhabited; planets with twice Earth’s mass and about 120% Earth’s radius are preferred. Planets with significant oceanic coverage but with shallower oceans, particularly along continental shelves, are assumed to be more conducive to life. Planets closer to the center of what was initially called the habitable zone should be more likely to be home to life than a planet toward the inner edge, like Earth. And planets around slightly lower-mass stars than our Sun with slightly denser atmospheres than Earth are deemed the most likely places for life to arise.
These assumptions are all highly questionable, however. Perhaps life is most likely to arise in freshwater lakes with volcanic activity beneath them — the hydrothermal fields hypothesis — rendering the oceanic coverage question irrelevant. Perhaps larger surface areas create more unstable, variable conditions across the planet, disfavoring life’s early emergence. Perhaps our notions of what constitutes a “habitable zone” are laughable. And perhaps higher-mass, more luminous stars, possessing more ultraviolet radiation, are more likely to give rise to life; perhaps K-type and M-type star systems are mostly barren.
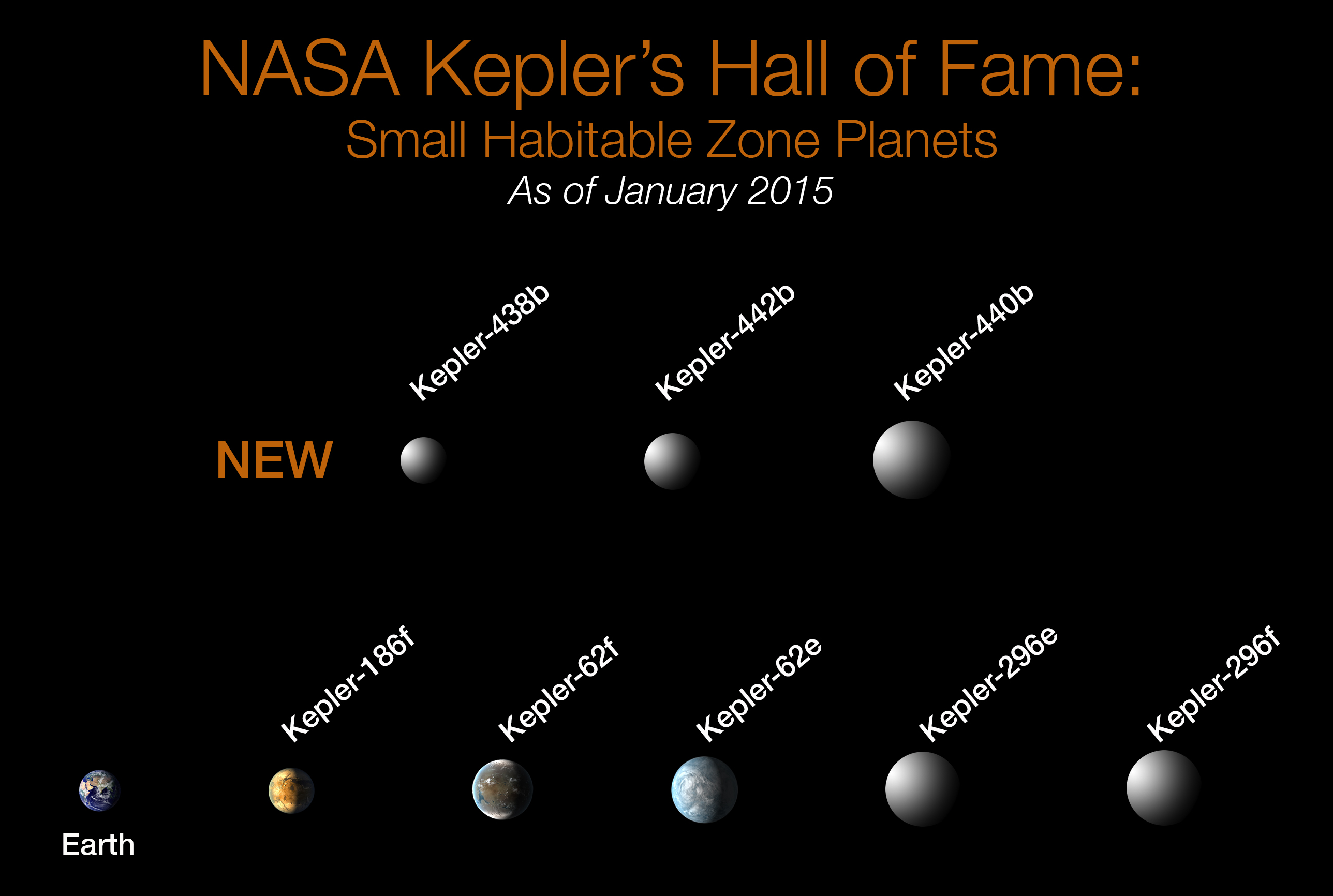
The eight most Earth-like worlds, as discovered by NASA’s Kepler mission: the most prolific planet-finding mission to date. All of these planets orbit stars smaller and less bright than the Sun, and all of these planets are larger than Earth, with many of them likely possessing volatile gas envelopes. Although some of them are called super-habitable in the literature, we don’t yet know if any of them have, or ever had, life on them at all.
There are many planets presently known that could be home to life. By the above criteria, some would be classified as super-habitable, but whether any of these worlds have life is vastly uncertain. Kepler-442b, for example, is frequently taken as the “most superhabitable” world known, but to assert that it’s more habitable than Earth is an absurdity with our present knowledge.
- It possesses 134% the radius of Earth and 230% the mass of Earth, placing it right on the border of having a volatile gas envelope around it.
- It orbits a K-type star that’s under 3 billion years old and has an average surface temperature of -40° C.
- The star it orbits has ~43% of the amount of heavy elements present in the Sun, indicating that it’s less enriched than our star system.
- And its atmospheric and ocean/land properties are completely unknown, having not been measured with present technology.
It may well be that Kepler-442b is a planet teeming with life. It may be the case that life has a greater diversity there, and that it has evolved to a more advanced stage more quickly than life did on Earth. But it’s also possible that there is not — and never was — life on that world, and that our present notions of habitability are completely wrongheaded and ill-informed. At this stage in the game, it makes sense to entertain possibilities and search for answers. To assert that we have them, however, is simply an exercise in unjustified arrogance.
https://bigthink.com/starts-with-a-bang/planet-more-habitable-earth/
2022-10-12 06:00:00Z
CAIiEG9zW6mKRm1ylrW85QpI9U0qMwgEKioIACIQt0sVd2IJWrv4unhvsO9ReCoUCAoiELdLFXdiCVq7-Lp4b7DvUXgw9JOCBw
Bagikan Berita Ini
0 Response to "Are there super-habitable planets compared to Earth? - Big Think"
Post a Comment